research
My previous research interests were focused around turbulent accretion discs as the environment of planet formation. This included numerical simulation of magnetohydrodynamic turbulence and its effects on the growth of planetesimals and protoplanets. Further interests include the interstellar medium and dynamo theory. The links below will direct you to a specific topic.
Core accretion
In collaboration with Neal J. Turner and Richard P. Nelson, I have been developing a global accretion disc model with an embedded planetary core. The disc is treated in full MHD with a self-consistent Ohmic resistivity derived from external ionization sources.
Disc model: Mass density in the disc, showing the opening of a gap in the disc, as well as the formation of spiral arms induced by the tidal torques emanating from the gravitational force of the planet.
With the help of adaptive mesh refinement techniques, we are able to resolve the magnetic field lines and gas flow in the close vicinity of the planet. This will allow us to identify potential bottle necks in the core-accretion paradigm of the formation of gas giant planets.
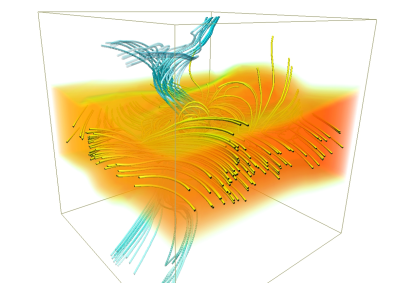
Embedded core: Close-up of magnetic field lines (blue) and streamlines (yellow) in the planet's immediate vicinity. The semi-transparent orange cloud shows the gas density distribution.
Planetesimal dynamics
Together with my previous supervisor, Prof. Dr. Richard P. Nelson, I was performing realistic computer simulations measuring the impact of magnetic turbulence on boulders and planetesimals of various sizes.
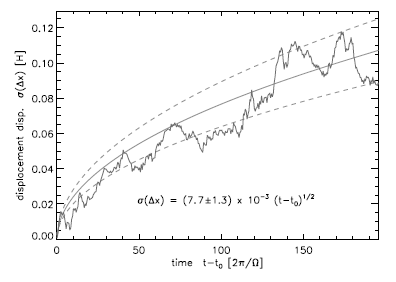
Random walk: Dispersion in the radial displacement Δx for a swarm of particles subject to the gas gravity alone. The time evolution follows a random walk as described by the given relation (from Nelson & Gressel 2010).
The currently accepted paradigm of how planets are formed is founded on the concept of dust coagulation and subsequent growth into larger building blocks, so-called planetesimals. This process happens in the gaseous nebula around a young star, referred to as a protoplanetary accretion disc. Such a disc, which can be seen in the excess infra-red emission detected in forming stars, is typically made-up of hydrogen and dust. In the context of planet formation, it is crucial to understand how particles are affected by this gaseous environment.
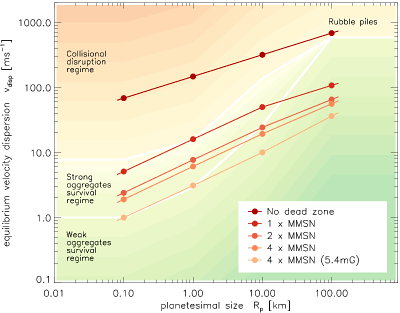
Velocity dispersion: Equilibrium velocity dispersions as function of body size for various studied models. In discs without dead zones, planetesimals are likely subject to collisional disruption during mutual impacts. In heavier discs, gas-drag damping is successively enhanced, and the velocity dispersion remains below the disruption threshold for sizes larger than 10km. In the case of weaker (i.e. Bz=5.4mG) magnetic fields, even smaller bodies may be safe.
Simulations of planetesimals embedded in fully turbulent, non-stratified disc models show that the velocity dispersion of km-sized bodies grows quickly and exceeds the threshold for catastrophic disruption. This raises important questions about the viability of planetesimal accretion in such turbulent discs. Our simulation of a fully turbulent, vertically stratified disc confirms this basic picture.
We find, however, that planetesimals which are embedded in a disc with a dead-zone whose vertical size is approximately two density scale-heights experience a substantially reduced stochastic forcing, being decreased by a factor of 10-20. It thus appears that dead-zones may provide an environment which is conducive to planet formation via planetesimal accretion.
related publications
- Gressel, O., Nelson, R. P., Turner, N. J. (2012), MNRAS 422, 1140: Dead zones as safe havens for planetesimals: influence of disc mass and external magnetic field
- Gressel, O., Nelson, R. P., Turner, N. J. (2011), MNRAS 415, 3291: On the dynamics of planetesimals embedded in turbulent protoplanetary discs with dead zones
- Nelson, R. P., & Gressel, O. (2010), MNRAS, 409, 1392: On the dynamics of planetesimals embedded in turbulent protoplanetary discs
Accretion disc dynamos
While the link between the turbulence amplitude and the stochastic forcing of embedded solids is now relatively well understood, the true physical magnitude of the magnetic fields and their efficiency in driving the turbulence remain unresolved. I believe that dynamo theory is a valuable tool in investigating the mechanism that ultimately leads to the saturation of the magnetic instability (known as MRI). This is a key requirement in obtaining save limits on the turbulence amplitude and hence on the prospects of growing planet-sized objects.
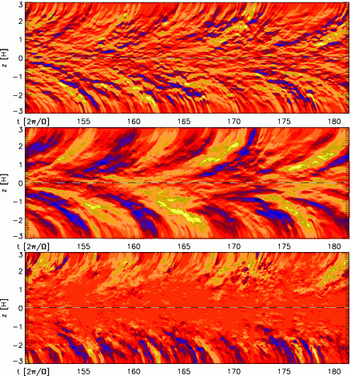
Butterfly diagram (simulation): Space-time evolution of the horizontally averaged radial field, azimuthal field, and magnetic α effect. The colour coding is normalized by the vertical rms amplitude at any given time (from Gressel 2010).
Local shearing box simulations of stratified magnetorotational turbulence exhibit cyclic field patterns which propagate away from the disc mid-plane (see figure above). A common explanation for this is magnetic buoyancy. The flow is, however, found to be buoyantly stable below one scaleheight, necessitating an alternative explanation in this region. I conducted and analysed direct numerical simulations to explain the observed behaviour by means of a mean-field description.
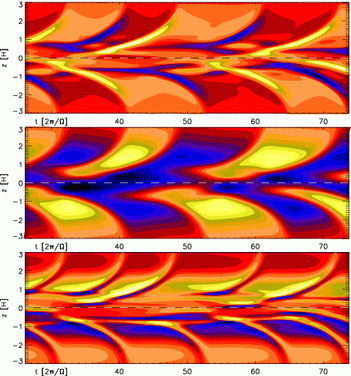
Butterfly diagram (model): Same as above, but for a simplified 1D mean-field model. The temporal evolution of α bears some similarity with its counterpart in the direct simulations (see figure above). There seems to exist a doubling of the cycle frequency in α, and the related pattern is likely related to a phase shift between the radial and azimuthal field (from Gressel 2010).
related publications
- Gressel, O. (2010), MNRAS, 405, 41: A mean-field approach to the propagation of field patterns in stratified magnetorotational turbulence
- Latter, H. N., Fromang, S., & Gressel, O. (2010), MNRAS, 406, 848: MRI channel flows in vertically stratified models of accretion discs
Interstellar turbulence
My PhD work was concerned with high-Mach-number interstellar turbulence driven by expanding shells from SNe. Our model of the turbulent ISM included various physical effects such as radiative cooling, thermal conduction and localised injection of thermal energy modelling the SNe. The aim of the project was to identify the role of realistically driven turbulence on the amplification of magnetic fields within the ISM.
ISM turbulence: This video is produced from a box simulation of supernova-driven interstellar turbulence. The colour-coded representation of the gas pressure on the left illustrates the vigorous forcing. The effect on the magnetic field is shown on the right. Because the turbulence is homogeneous, there is no net helicity produced.
My thesis results confirm the theoretically predicted dynamo effect and the associated transport processes. Beyond measuring mean-field coefficients, I was the first to demonstrate that turbulence driven by SNe alone leads to a rapid exponential amplification of the mean magnetic field on a time scale of only hundred million years.
Stratified ISM: Similar to above, but now including the vertical structure of the galactic disc. The four panels show, number density, gas pressure, temperature, and magnetic field. Note how the rightmost panel slowly changes its colour to lighter green, indicating the overall field amplification. This amplification is due to a helical dynamo resulting from the inhomogeneity of the turbulence.
In an ongoing collaboration with Udo Ziegler and Detlef Elsnter, I will support a PhD student at the AIP modifying our existing ISM model to include anisotropic cosmic ray diffusion. Cosmic rays provide a potentially important effect by supporting buoyant field instabilities, which can drive a Parker-type dynamo (Hanasz et al., 2004, ApJ 605, 33).
related publications
- Gressel, O., Bendre, A., Elstner, D. (2013), MNRAS (in press): On the magnetic quenching of mean-field effects in supersonic interstellar turbulence
- Gressel, O. (2009), A&A, 498, 661: A Field-length based refinement criterion for adaptive mesh simulations of the interstellar medium
- Gressel, O., Elstner, D., Ziegler, U., Rüdiger, G. (2008), A&A, 486, L35: Direct simulations of a supernova-driven galactic dynamo
- Gressel, O., Ziegler, U., Elstner, D., Rüdiger, G. (2008), AN, 329, 619: Dynamo coefficients from local simulations of the turbulent ISM