Horizon 2020 - ERC Starting Grant
"First-principles global MHD disc simulations:
Defining
planet-forming environments
in early solar systems"
The aim of my research project is to create the most realistic computer simulations of protoplanetary discs of gas and dust, thus defining the environment that shapes the early development of planetary systems.
![]() |
Project overview
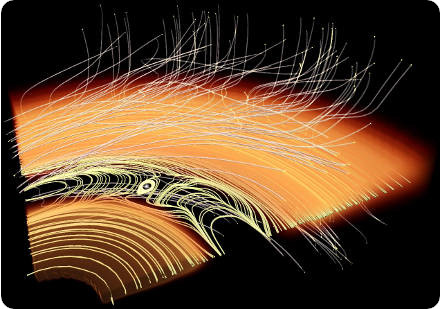
In their role as planet nurseries, protoplanetary discs are of key interest to planet formation theory. Their dynamical, radiative and thermodynamic properties critically define the environment for embedded solids: dust grains, pebbles and planetesimals. In short, the building blocks of planet formation. The discs' dynamics and structure in turn depend critically on the influence of magnetic fields that couple to tenuously ionised and low-density regions. Being comparatively cold and dense, the ionisation state of the disc plasma is dominated by external far-UV, X-Ray, and cosmic-ray radiation, leading to a layered vertical structure - with turbulent surface layers and a magnetically-decoupled midplane. This classic dead-zone picture is now turned upside-down by previously ignored microphysical effects.
Highlight: Magnetocentrifugal winds
Protoplanetary disks accrete onto their central T Tauri star via magnetic stresses. When the effect of ambipolar diffusion is included, and in the presence of a vertical magnetic field, the disk remains laminar in its planet-forming inner region, and a magnetocentrifugal disk wind forms that provides an important mechanism for removing angular momentum. We have performed global MHD simulations where the time-dependent gas-phase electron and ion fractions are computed under FUV and X-ray ionization with a simplified recombination chemistry.
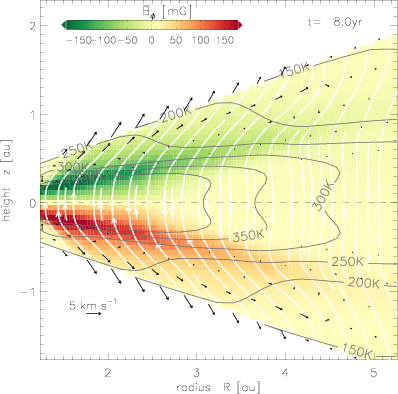
Radiative-magnetic wind model: Detail from a proof-of-concept global MHD simulation of a PPD with ambipolar diffusion, radiative transfer and stellar irradiation, showing the magnetic field strength (colour), velocity arrows (black) and magnetic field lines (white), and iso-contours of the radiation temperature (grey).
To investigate whether the mass loading of the wind is potentially affected by the limited vertical extent of our existing simulations, we attempt to develop a model of a realistic disk atmosphere. To this end, by accounting for stellar irradiation and diffuse reprocessing of radiation, we aim at improving our models towards more realistic thermodynamic properties.
related publications
- Gressel, O. (2016), arXiv:1611.09533:
“Toward realistic simulations of magneto-thermal winds from weakly-ionized protoplanetary disks” - Brinch, C., Jørgensen, J. K., Hogerheijde, M. R., Nelson,
R. P., Gressel, O. (2016), ApJ 830, L16:
“Misaligned Disks in the Binary Protostar IRS 43”
Highlight: Jupiter acting as a dust barrier
Aerodynamic theory predicts that dust grains in protoplanetary disks will drift radially inward on comparatively short timescales. In this context, it has long been known that the presence of a gap opened by a planet can alter the dust dynamics significantly. Lead by PhD student Philipp Weber, we have carried out a systematic study employing long-term numerical simulations aimed at characterizing the critical particle-size for retention outside a gap as a function of particle size and for various key parameters defining the protoplanetary disk model.
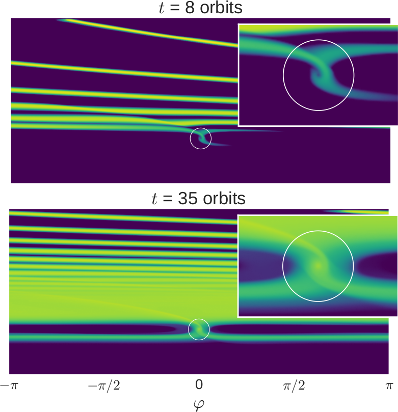
Dust stream around an embedded planet: Surface density of ten micron-sized dust that was initialized in a single azimuthal grid cell outside the planet's location. The panels show the distribution of dust after 8, and 35 orbits, respectively, in a frame that is co-rotating with the planet, and the circle illustrates the planet's sphere of influence.
In agreement with previous work, we find that the permeability of the gap depends both on dust dynamical properties and the gas disk structure: while small dust follows the viscously accreting gas through the gap, dust grains approaching a critical size are progressively filtered out.
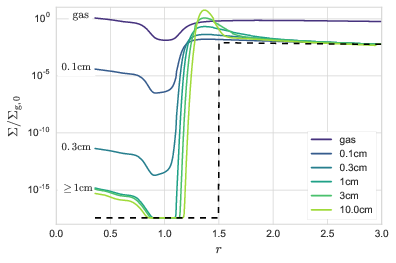
Gap permeability as a function of particle size: The figure shows radial profiles of the dust surface density, Σd normalized to the initial gas surface density, Σg,0. Particles larger in size than about a tenth of a centimeter begin to aerodynamically decouple from the gas flow, and, as a result, become significantly depleted in the inner system.
We introduce and compute a depletion factor (see figure above) that enables us to quantify the way in which higher viscosity, smaller planet mass, or a more massive disk can shift this critical size to larger values. Our results indicate that gap-opening planets may act to deplete the inner reaches of protoplanetary disks of large dust grains, potentially limiting the accretion of solids onto forming terrestrial planets.
related publications
- Weber, P., Benítez-Llambay, P., Gressel, O., Krapp, L.,
Pessah, M. E. (2018), ApJ 854, 153:
“Characterizing the Variable Dust Permeability of Planet-induced Gaps” - Haugbølle, T., Weber, P., Wielandt, D. P., Benítez-Llambay, P., Bizzarro, M., Gressel, O., Pessah, M. E. (2019), AJ 158, 55: “Probing the Protosolar Disk Using Dust Filtering at Gaps in the Early Solar System”
Highlight: Self-organization with Hall-MHD
Imaging of dust continuum emitted from disks around nearby protostars reveals diverse substructure. In recent years, theoretical efforts have been intensified to investigate in how far the intrinsic dynamics of protoplanetary disks (PPDs) can lead to such features. Turbulence in the realm of non-ideal magnetohydrodynamics (MHD) is one candidate for explaining the generation of zonal flows which can lead to local dust enhancements.
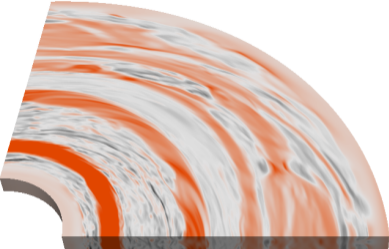
Spontaneous self-organization of magnetic flux: In this snapshot from a Hall-MHD simulation, the turbulent fluctuations created by the magneto-rotational instability have undergone an extended phase of self-organization leading to zonal bands and vortices. In our case, four bands of vertical magnetic flux are obtained.
In an effort led by PhD student Leonardo Krapp, we consider combinations of vertical and azimuthal initial net flux and perform 3D non-ideal MHD simulations aimed at studying self-organization induced by the Hall effect in turbulent PPDs. We include dust grains, treated in the fluid approximation, in order to study their evolution subject to the emerging zonal flows. In the regime of a dominant Hall effect, we robustly obtain large-scale organized concentrations in the vertical field that remain stable for many orbits.
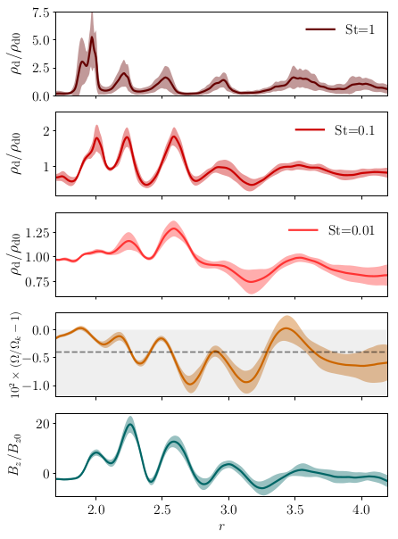
Accumulation of dust into rings: Grains of various sizes (top three panels) concentrate at locations in the disk, where the flow deviates from its sub-Keplerian equilibrium profile (4th panel), related to the accumulation of magnetic flux (5th panel). The aerodynamic coupling of the particles is characterized by their so-called Stokes number, St, describing their relative coupling to the gas.
Our research has, moreover, found that including a moderately strong net-azimuthal magnetic flux can significantly alter the dynamics, partially preventing the self-organization of zonal flows. However, in all cases, we demonstrate that the emerging features are capable of accumulating dust grains for a range of Stokes numbers.
related publications
- Krapp, L., Gressel, O., Benitez-Llambay,
P., Downes, T., Mohandas, G. & Pessah, M. (2018), ApJ 865, 105:
“Dust segregation in Hall-dominated turbulent protoplanetary disks”
Highlight: Streaming instability for particle-size distributions
The so-called streaming instability is thought to play a central role in the early stages of planet formation by enabling the efficient bypass of a number of barriers hindering the formation of planetesimals. It provides an incredibly fast mechanism to jump more or less directly from pebble-sized objects to bodies the size of asteroids, or even the Moon. Because of its near-magical efficiency, many researchers believe that it offers a compelling shortcut to outrun the clock set by the disks lifetime.
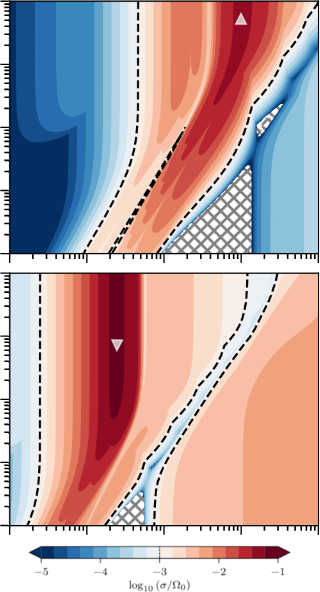
Growth rate of the most unstable mode for the multi-species (here, N=16) streaming instability as a function of radial/vertical wave number. Results are shown for two specific number-density distributions extending to moderate grain sizes (upper panel) and large grain sizes (lower panel), bot with power-law of index q=-3.5 in the particle-size and equal dust-to-gas mass ratio of unity.
The idea has now been around for a bit over a decade, and for matters of convenience and tractability, most theoretical and computational studies have focused on mixtures comprised of gas and a single dust species, typically representing the pebble-sized progenitors, which mark the barrier of conventional growth mechanisms. We have now developed an advanced framework to study this important mechanism for the more realistic case of a continuous dust-size distribution.
Exploiting this new framework, the team headed by PhD student Leonardo Krapp, present the first study exploring the efficiency of the linear streaming instability when a particle-size distribution is considered. They find that, for a given dust-to-gas mass ratio, the multi-species streaming instability grows on timescales much longer than those expected when only one dust species is involved. These findings challenge — and potentially jeopardize — the express-way shortcut that the original streaming instability had so elegantly provided.
related publications
- Benítez-Llambay, P., Krapp, L., Pessah, M. E. (2019), ApJS
241, 25:
“Asymptotically Stable Numerical Method for Multispecies Momentum Transfer: Gas and Multifluid Dust Test Suite and Implementation in FARGO3D” - Krapp, L., Benítez-Llambay, P., Gressel, O., Pessah, M. E. (2019), ApJ 878, L30: “Streaming Instability for Particle-size Distributions”
Highlight: Dust signatures of planet migration
Planet migration is a key ingredient to our comprehension of early planetary evolution. Unfortunately, theoretical predictions strongly rely on assumed initial conditions, and thus describing the migration behaviour is still challenging and further hindered by poorly constrained knowledge about physical conditions in the environment where it takes place.
The positions at which planets are detected after the disk's dispersal do not necessarily shed light on these questions, since planets are expected to change their orbital elements within the gaseous phase of the protoplanetary disk.
In this project, we propose a method to possibly detect planet migration from highly resolved, state-of-the-art observations, using a large-baseline configuration of the Atacama Large Millimeter Array (ALMA). For this, we exploit that a medium mass planet creates three closely spaced rings in the dust component within a low-viscosity environment.
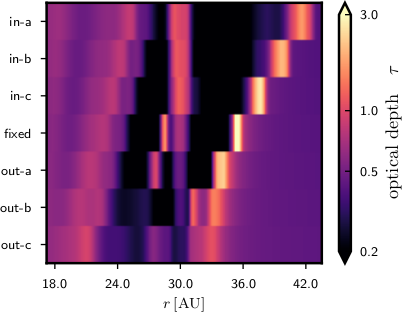
Theoretical models of dust rings showing the changing radial spacing of dust accumulations for seven cases of varying migration rate (top three panels: inward, center panel: no migration, bottom three panels: outward at increased speed).
By studying the influence that a migrating planet has on this structure, we realise that the mutual separation of the three rings is mostly dependent on the planet's state of migration: if the planet is not migrating, the inner and outer rings appear to be almost equally spaced from the central one. An inwards migrating planet creates an asymmetry that shifts the central ring closer to the ring located inwards of the planet, an outwards migrating planet creates the opposite effect.
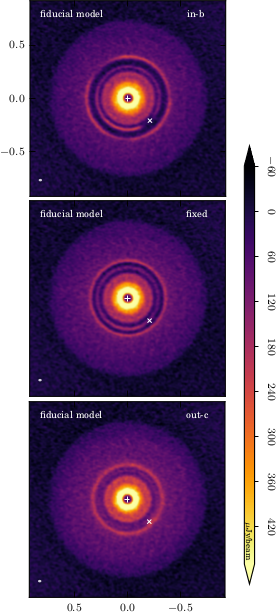
Synthetic continuum maps illustrating how the different cases with varying radial spacing of dust accumulations may be detected in high-resolution ALMA dust-continuum images.
With the simulation models, we perform subsequent radiative transfer calculations and image synthesis to show that the different shapes of the triple ring structure can in principle be distinguished from each other with modern instruments such as ALMA. These results motivate future observations of promising protoplanetary disk candidates, in order to find out whether and how planets migrate.
related publications
- Weber, P., Pérez, S., Benítez-Llambay,
P., Gressel, O., Casassus, S., Krapp,
L. (2019), arXiv:1909.01661:
“Predicting the observational signature of migrating Neptune-sized planets in low-viscosity disks”
Highlight: Thermally assisted MHD winds
Outflows driven by large-scale magnetic fields likely play an important role in the evolution and dispersal of protoplanetary disks, and in setting the conditions for planet formation. We extend our 2-D axisymmetric non-ideal MHD model of these outflows by incorporating radiative transfer and simplified thermochemistry, with the twin aims of exploring how heating influences wind launching, and illustrating how such models can be tested through observations of diagnostic spectral lines.
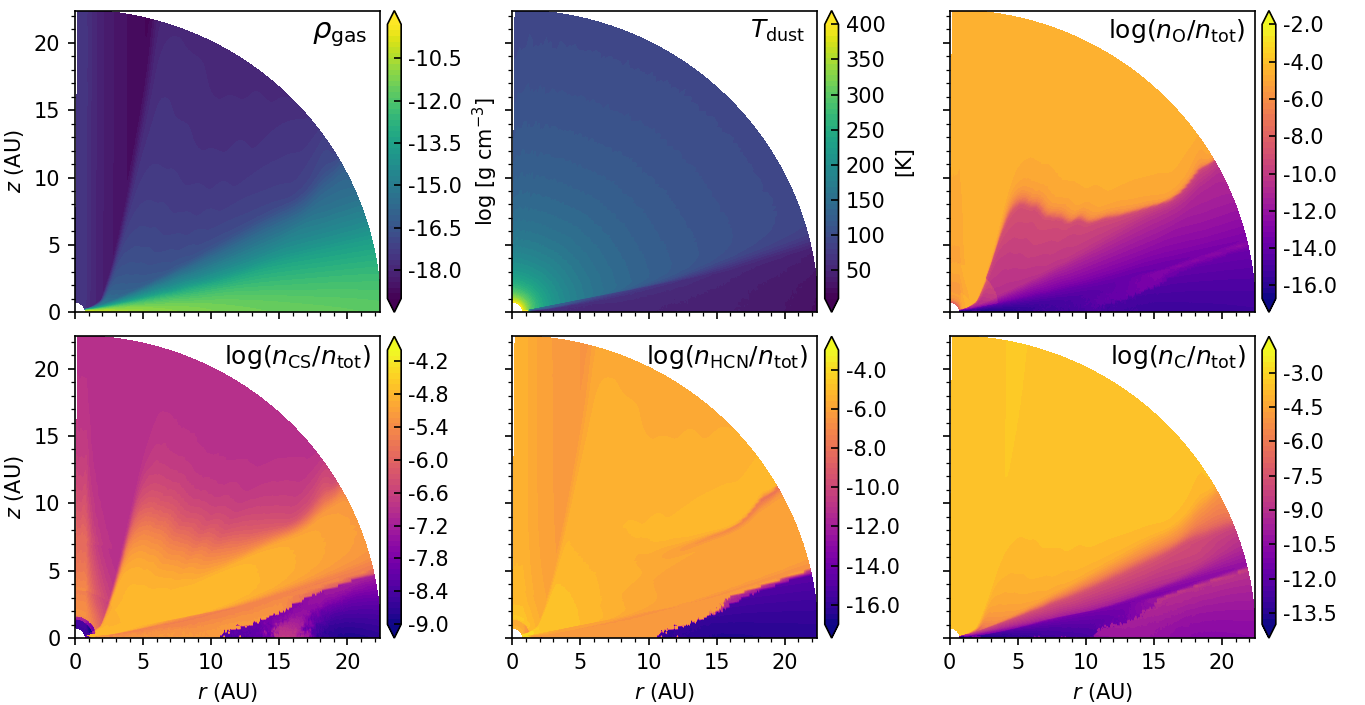
Chemical Abundance Maps Overall gas density, plus the dust temperatures and chemical abundances of select species (relative to ntot = nH+ 2nH2) obtained by post-processing the model.
Our model disks launch magnetocentrifugal outflows primarily through magnetic tension forces, so the mass-loss rate increases only moderately when thermochemical effects are switched on. For typical field strengths, thermochemical and irradiation heating are more important than magnetic dissipation. We furthermore find that the entrained vertical magnetic flux diffuses out of the disk on secular timescales as a result of non-ideal MHD.
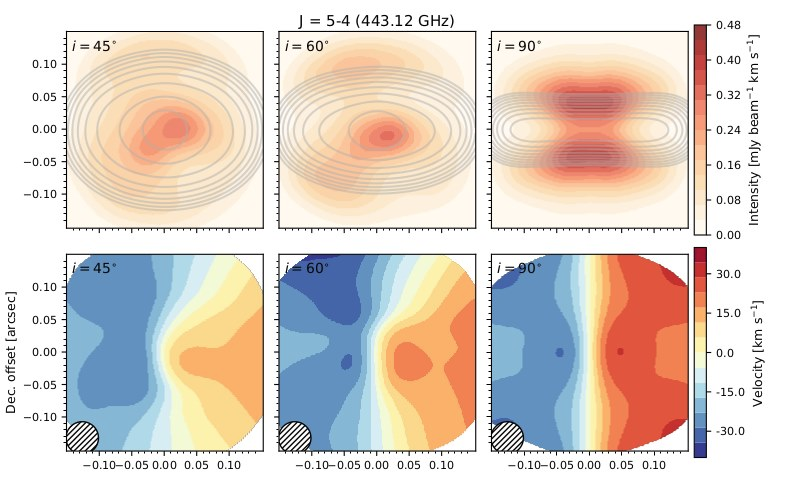
Synthetic moment map of the J=5-4 (443.12 GHz) transition of the HCN molecule. The top two (bottom) rows show the moment-0 and moment-1 maps at inclinations of 45°, 60°, and 90°.
Through post-processing line radiative transfer, we demonstrate that spectral line intensities and moment-1 maps of atomic oxygen, the HCN molecule, and other species show potentially observable differences between a model with a magnetically driven outflow and one with a weaker, photoevaporative outflow. In particular, the line shapes and velocity asymmetries in the moment-1 maps could enable the identification of outflows emanating from the disk surface.
related publications
- Gressel, O., Ramsey, J. P., Brinch, C., Nelson, R. P., Turner, N. J., Bruderer, S. (2020), arXiv arXiv:2005.03431:
“Global Hydromagnetic Simulations of Protoplanetary Disks with Stellar Irradiation and Simplified Thermochemistry”
![]() |
This project has received funding from the European Research Council (ERC) under the European Union's Horizon 2020 research and innovation programme (grant agreement No 638596). |